Sterile Neutrino Dark Matter Detection
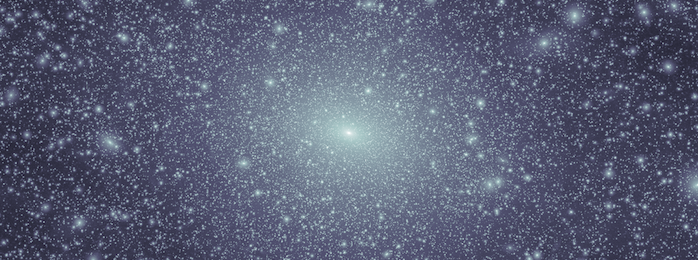
Indirect detection in X-rays
Indirect detection of dark matter proposes to exploit the standard model signatures left from dark matter interactions or decays, if these exist. Many dark matter models propose that dark matter either decays, self-interacts, or can de-excite in ways which produce standard model particles. Since we are surrounded by the Milky Way's dark matter halo, some experiments look for an excess in the abundance of rare particles in cosmic rays (such as anti-protons or anti-deuterons). Other experiments look for excess photon emission from the Milky Way, dwarf spheroidal and other galaxies, and galaxy clusters.
We concentrate on models which produce mono-energetic photons in the X-ray range. Sterile neutrinos are the best-known model for this emission, as we know that neutrinos have mass, and an easy way to fit massive neutrinos into the standard model is by postulating the existence of right-handed or sterile neutrinos. More than one species of sterile neutrino could exist, and if one of these has a mass between 1-100 keV (in some theories it could be even heavier), it could be the dark matter. These keV sterile neutrinos would decay (with a very long time constant) into a standard model neutrino and an X-ray of energy equal to half the sterile neutrino mass. Thus we could detect dark matter in the Milky Way by looking for unexplained all-sky X-ray lines.
Although we focus on sterile neutrino models, many other models with axion-like particles, exciting dark matter, gravitinos, moduli, WIMPs, etc. can produce X-ray line emission. Limits on X-ray line emisson place constraints on all these models, and if a line were seen, careful mapping of the signal and comparisons with direct detection and collider results would be needed to begin to disentangle which of these models was the correct one.
X-ray indirect detection requirements
There are various strategies for looking for X-ray emitting dark matter. With current X-ray observatories such as XMM-Newton and Chandra, their small field of view means they obtain better signal-to-noise when observing objects with large expected emission from dark matter, such as the centers of galaxy clusters. The Milky Way galactic center offers the largest signal, but has very complicated and bright backgrounds. We are designing observations using sounding rockets, which allow us to maximize the Grasp or Etendue, defined as Field of View x Effective Area (FOV×Aeff) of the system. The high-energy resolution of our detectors, coupled to their grasp, allow us to obtain better sensitivity to keV dark matter lines with a 300 second observation than megasecond observations with XMM-Newton.